Photo by Simi Luft
It’s time for a critical zone coming out party.
The critical zone—Earth’s thin living skin, which spans from the top of the canopy to the bottom of the groundwater—is still unfamiliar to many scientists.
More than a concept, it’s an interdisciplinary endeavor that draws on multiple fields to piece together the complicated interactions between water, air, life, rock, and soil that support terrestrial life. By understanding and modeling these relationships and how they evolve, scientists can predict how human activities threaten these necessary, life-sustaining systems.
Critical zone science began almost 2 decades ago and developed in the United States through a network of observatories funded by the National Science Foundation (NSF). Now, as a new generation is applying critical zone science to novel questions and sites, the discipline has finally come of age.
The Birth of a New Field
Gail Ashley, a professor emerita at Rutgers University, first coined the term critical zone in a prophetically titled 1998 abstract for the Geological Society of America meeting, “Where Are We Headed?” “I called it the critical zone because it’s critical for life,” she said. “Also, it’s critical to know more about it because of the potential for damaging it.”
A sedimentary geologist, Ashley spent most of her career reconstructing the ancient paleo–critical zone of Olduvai Gorge in Tanzania, one of the richest paleoanthropology sites in the world. Her team uncovered evidence of wetlands and freshwater springs that likely supported hominins living at the site more than a million years ago.
The concept of critical zone science gained traction through a 2001 National Academies report, to which Ashley contributed, that recommended the creation of natural observatories for studying the critical zone.
Susan Brantley, a geochemist at Pennsylvania State University, was a driving force in developing critical zone research in the United States. “In the early 2000s, hydrology was funded separately from geochemistry, was funded separately from geomorphology,” said Brantley. “A lot of us were starting to talk about how we…should be looking at the Earth’s surface as one thing and bringing all those subdisciplines together.” In 2003, Brantley led a team that hosted workshops, wrote white papers, and held town halls at national and international meetings to recruit Earth scientists interested in how biological, physical, and chemical processes shape Earth’s surface.
Brantley and her colleagues proposed various sites for laboratories dedicated to the study of the critical zone, and in 2006, NSF put out the call for interdisciplinary watershed-scale projects that would “advance our understanding of the integration and coupling of Earth surface processes as mediated by the presence and flux of fresh water.” The Critical Zone Observatories (CZOs), the first long-term sites supported by NSF’s Directorate for Geosciences, were born.
Data: The Language of Science
In 2007, NSF awarded 5-year grants to establish three CZOs, all, coincidentally, in forest watersheds. Brantley was a co–principal investigator at the Susquehanna Shale Hills site, an eastern forest on weathering shale bedrock in central Pennsylvania. Sites in California’s southern Sierra Nevada and one in the Colorado Rockies also received funding. The American Recovery and Reinvestment Act of 2009 provided funding for three more observatories, with a wider variety of river basins. Finally, in 2013, the number of CZOs grew to nine, with the closure of one site and four additions. Long-term funding was critical for understanding how the critical zone functions normally and how it responds to disturbances, like fires or floods.It took a few years for collaborators from different fields to understand each other’s science enough that they could help each other out, “and then we really began to be able to do truly interdisciplinary science.”“One of the challenges early on was just finding a vocabulary,” said Timothy White, a geologist at Penn State University and the national office director of the CZOs. It took a few years, he said, for collaborators from different fields to understand each other’s science enough that they could help each other out, “and then we really began to be able to do truly interdisciplinary science.”
“From the beginning, it was also about data, how to collect all of these data,” said NSF program director Enriqueta Barrera, who nurtured the program from its infancy.
Ultimately, data and new technologies became common tongues in critical zone science. Advanced techniques in isotope geochemistry helped scientists track the movement of elements through the critical zone, estimate the size of fluxes, and calculate the speed of chemical processes on timescales ranging from minutes to millennia. Through remote sensors, researchers monitored weather, soil moisture and temperature, sap flow, and snow depths from the comfort of their labs. Using lidar, they mapped changes to the landscape from planes. The bounty of data were integrated into numerous geochemical, hydrological, and climate models.
Other countries began to replicate the CZO system. In collaboration with CZO scientists, researchers have established observatories around the world, on every continent except Antarctica. In the United States, the Department of Energy modeled some long-term monitoring sites after CZOs, and many critical zone scientists worked independent of the observatories.
How Far Can You Push the Critical Zone?
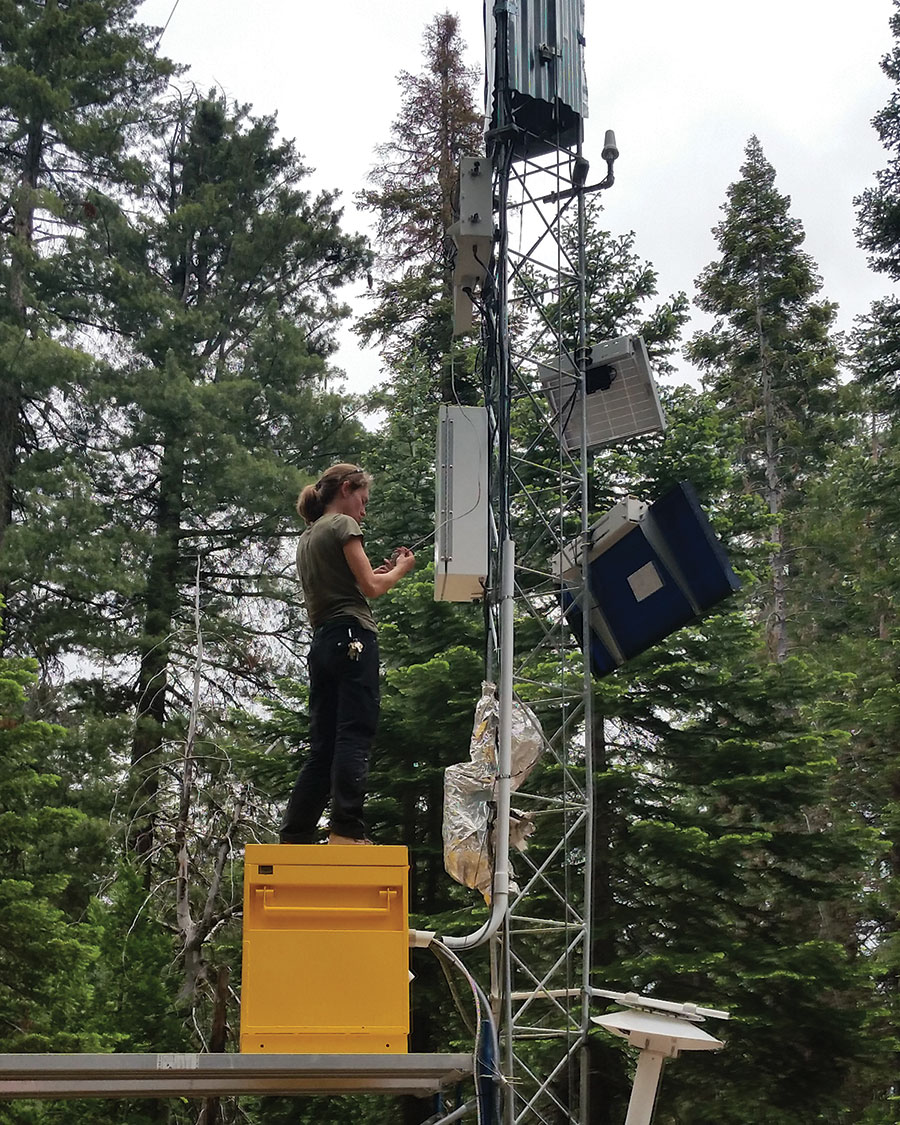
Since the field’s inception, critical zone scientists have shown how altering Earth’s surface and vegetation leads to subsurface impacts—changing water flow, minerals, and microbial life at surprising depths. Subsurface imaging and drilling have also shown considerable belowground variation and the ways in which that alteration influences surface conditions. These discoveries opened the door to more applied research and better land management practices.
For example, critical zone studies in California let researchers predict how much deep water, stored in pores in weathered bedrock, is available to forests during droughts. Scientists at the Southern Sierra and Eel River CZOs reported the amounts of root-accessible moisture available from year to year, which sustained plant life during the severe 2011–2015 drought. Nearly 150 million trees succumbed to the drought, mainly in the southern Sierra Nevada. These die-offs were a direct result of the gradual depletion of the root-accessible moisture by overstocked forests—caused by long-term fire suppression—according to research by Roger Bales, a hydrologist at the University of California, Merced, and colleagues at the Southern Sierra CZO.
Now Bales is making recommendations to the U.S. Forest Service and other land managers based on this work. “We can provide metrics that tell you where the forest is likely to suffer drought stress,” said Bales, “based not just on precipitation and temperature, which is what traditional drought indices do, but also factoring in over-year storage provided by long-term bedrock weathering.”
In the Midwest, at the trio of agricultural sites in the Intensively Managed Landscapes CZO in Illinois, Iowa, and Minnesota, researchers showed that industrial farming has changed the prairie landscape from one of transformation into one dominated by transport. The prairie ecosystem that evolved after glaciers from the last ice age receded functioned primarily by cycling nutrients in place and holding water in soil and groundwater (transformation). But with industrial farming, added fertilizer is partially taken up and harvested as grain, and the rest is transported away by water through altered surface pathways and subsurface drainage, ultimately fueling algal blooms in the Gulf of Mexico.
“There is a direct link between what we are finding from how these critical zones function and what we can do better in terms of ecosystem services,” said Praveen Kumar, a hydrologist at the University of Illinois and head of the Intensively Managed Landscapes CZO.
Growing the Critical Zone Community
A crucial outcome from the development of the CZOs is a new generation of Earth scientists trained to think within a multidisciplinary framework. As of 2018, the CZOs had trained more than 300 graduate students and more than 100 postdocs. At least 40 have gone on to become assistant professors.Critical zone science is “this ability to get people to bring in different data sets and different mindsets and perspectives to really challenge the data about what we’re seeing.”One former trainee is Pamela Sullivan, an ecohydrologist at Oregon State University. As a postdoc at the Shale Hills CZO, she used monitoring wells to study a series of weathering processes that occurred as water flowed across the small catchment. Now she studies how factors like plant roots, bedrock fracture networks, or a shift from snow to rain alter soil structure and subsurface water flow, to better inform climate models. Working in a multidisciplinary team at the CZO was a “transformative experience,” said Sullivan. “It’s this ability to get people to bring in different data sets and different mindsets and perspectives to really challenge the data about what we’re seeing.”
Sullivan acknowledges, however, that some have felt left out of critical zone science because they were not affiliated with an observatory. To encourage collaboration, she serves as a co–principal investigator on a Critical Zone Research Coordination Network focused on synthesizing data and models to investigate the evolution of the critical zone and how it will respond to future human impacts. The group facilitates networking to advance research within the community.
As critical zone science expands, researchers say the field has finally matured, although “it’s not an exaggeration to think of the critical zone as a new science,” said William Dietrich, head of the Eel River CZO. “Once you see the thing, you can’t walk away from it…. I think the critical zone is here to stay.”
The End of the Observatory Era?
Despite, or perhaps because of, the success of the CZO program, NSF will conclude its funding of the program in 2021. NSF program director Richard Yuretich is spearheading a new program that focuses on testing principles across a cluster of sites instead of comprehensive studies at a single observatory. Ideally, the data—aided by high-performance computing—will be integrated into comprehensive models of critical zone processes across diverse environments. The Consortium of Universities for the Advancement of Hydrologic Science Inc. (CUAHSI), a nonprofit that supports interdisciplinary water science, will serve as the critical zone hub. CUAHSI and collaborators will coordinate activities among the clusters, support training and synthesis activities, and provide cyberinfrastructure for collaborative modeling and for sharing and archiving data.
The shift was unwelcome news to some researchers. “I think it’s going to be a great opportunity for the younger generation,” said White, “but I think it was a shame that the literally tens of millions of dollars that were invested into the observatory network that we have is mostly being cast aside.”
NSF has funded nine critical zone network clusters, which began in September 2020. Many clusters include locations at existing CZOs or long-term field sites with legacy data, but are led by a new wave of early-career scientists. “We have a whole new generation of scientific researchers who now have this in their DNA,” said Yuretich. “The new thematic cluster and coordinating hub network is designed to capitalize on these individuals and bring them into the forefront of designing new research in the critical zone.”
Now free from the constraints of the observatories, scientists can ask questions that transcend location. Teams are investigating how the coastal critical zone responds to rising sea levels and saltwater intrusion, what big data can reveal about how the critical zone recovers from disturbances, the limits of human interference, and the many functions of dust as it travels from its source in the Rocky Mountains to the deserts of southern Nevada.
Forecasting the Future of Critical Zone Science
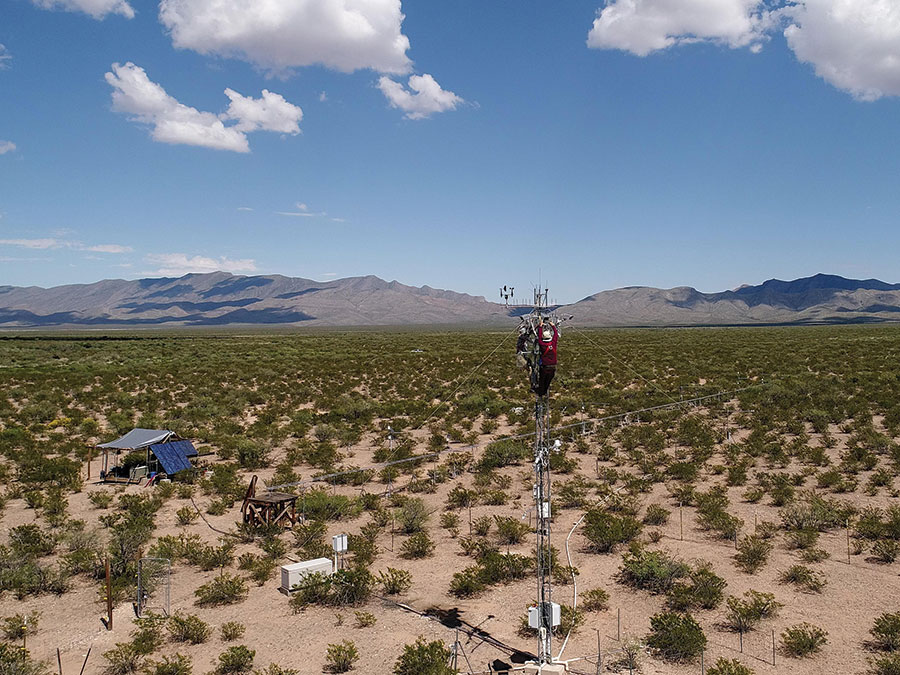
The new program will also help to deepen understanding of critical zone function beyond alpine watersheds. By graduating to a wider variety of environments, the ultimate goal is for researchers to construct more comprehensive models of critical zone processes worldwide.
One new critical zone network team will focus its operations in the Chihuahuan Desert, which receives about 20 centimeters of water each year, mostly during the July monsoon season. Dryland sites like this have been underrepresented in previous critical zone work. Lixin Jin, a geochemist at the University of Texas at El Paso and a former postdoc at the Shale Hills site, leads the project. She is fascinated by the desert environment, despite growing up in frigid Jilin Province, the Minnesota of China. What she discovers with her colleagues, including co–principal investigator and fellow University of Texas at El Paso geochemist Lin Ma, may have important implications for the future of the Chihuahuan and other dryland environments. These cover about 40{464181316c28bea4db76e6a38127b032d62ba553e1a210ad2f4f889997fffc93} of Earth’s landmass and support more than 2 billion people.
Unlike tropical or temperate environments, where precipitation leaches minerals from rocks and soil, limited water in the desert concentrates minerals into a cement-like layer called caliche. This water-impermeable layer builds up over thousands of years. “If you poke it with your finger, it’s as hard as rock,” said Jin. “We’re trying to understand how this unique architecture will govern how the ecosystem and water function in the critical zone.”
To see whether precipitation reaches the subsurface, the team will drill wells more than 100 meters deep to access El Paso’s freshwater source. Despite multiple hydrology studies, the city does not know the size of its reserves or whether surface water is refilling the aquifer. “To be honest, we don’t know what happens to the loose water,” said Ma. “But some of the water may make it all the way down.”
For comparison, the team is looking at younger soils along the Rio Grande, where farmers grow pecans, chilis, and alfalfa. Irrigation from the river carries a lot of minerals, and in less than a century of agriculture, farmlands have already been shown to accumulate salt, which stresses crops. Critical zone researchers will examine how irrigation alters the fate of surface water—whether it enters plants, the atmosphere, or the subsurface—and whether irrigation contaminates drinkable groundwater by mobilizing salt, nutrients, and trace metals.
Jin and her collaborators are also excited to bring a dryland critical zone curriculum to local classrooms and to recruit students from the local Latino population. “Especially in the geology field, or Earth science in general, I just don’t think we have good representation of the minority groups,” said Jin. “We really want to prepare and train the next generation of scientists.”
The ultimate goal of this and other critical zone work is to develop models that transcend the boundaries of individual research sites so we can “Earthcast,” or predict how Earth will respond to a changing climate and more intensive land use.
“We have forecast the weather,” said Jin. “But can we Earthcast the critical zone?”